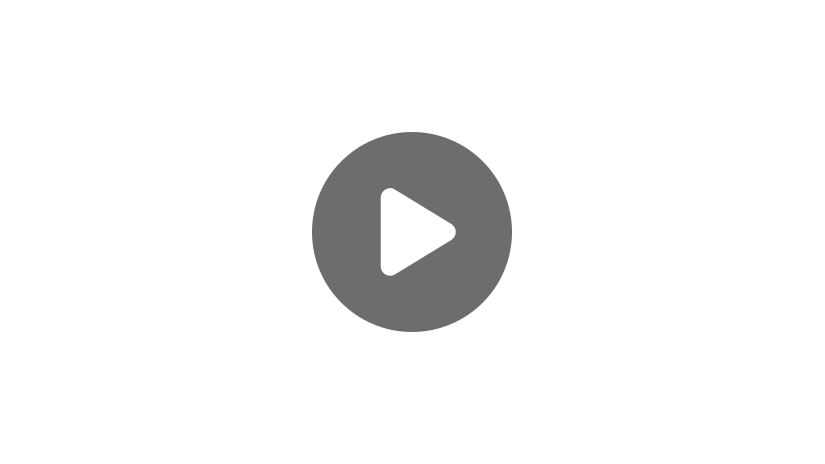
Hi, and welcome to this video on the chemical reaction process!
Put simply, chemical reactions are the breaking and forming of bonds, which typically entail the rearrangement of electrons (this definition excludes nuclear reactions). We’ll start by looking at the chemical equation (the standard notation for a reaction), then move on to discuss the more complex concepts of direction and speed.
Reactants, Products, and Balancing
Throughout this video, we’re going to use the reaction of hydrogen and oxygen to produce water as an example. So, let’s begin with the notation used to represent this.
This is the balanced chemical equation for the reaction:
The reactants, hydrogen and oxygen, are on the left side of the arrow and the product, water, is on the right side. The arrow can be read as, “produces”, so this equation is read as “two hydrogen molecules plus one oxygen molecule produces two molecules of water.” The arrow points in the direction desired by the chemist, but we’ll discuss later; the way the reaction actually proceeds is a more complicated matter.
You’ll notice that hydrogen and water both have coefficients of two. This indicates that two molecules of hydrogen gas are required to react with one oxygen molecule to produce two water molecules. Consider if we had written the equation without coefficients.
Notice how on the left side of the reaction, there are two hydrogen atoms and two oxygen atoms in total but on the right side, there are two hydrogen atoms but only one oxygen atom. This is an unbalanced reaction. It violates the principle of mass conservation because an oxygen atom has seemingly disappeared.
In theory, we could fix this by adding a lone oxygen atom, like this:
However, a single oxygen atom on its own is very unfavorable, so much so that this would never happen under normal conditions. Instead, if we were to just provide an additional molecule of hydrogen, we could form two molecules of water.
Now, there are four hydrogens and two oxygens on the left and right sides of the arrow, so our equation is balanced and no longer in violation of a fundamental law of science. Phew!
Phase Notation
Now, it’s often useful to know what phase our reactants and products are in. This not only helps us visualize the reaction, but also will be useful when trying to control the direction and speed of the reaction. We do this by including a g, l, or s after the substance to indicate that it is in the gas, liquid, or solid phase, respectively.
Additionally, you will see some substances listed as “aq,” which stands for aqueous, signifying that it is dissolved in water. For example, the chemical equation for sodium chloride dissolving in water specifies that the solid salt is converted to solvated ions.
Equilibrium
Now that we understand how to represent a reaction, let’s return to the arrow. Unfortunately, just drawing the arrow in a specific direction doesn’t mean all of the reactants will become the desired products. In fact, this scenario is quite unusual.
Instead, without intervention and given enough time, most reactions reach an equilibrium state with constant concentrations of both the reactants and the products. Keep in mind that this does not mean that they are no longer reacting, but only that the forward reaction is happening at the same rate as the reverse reaction.
Consequently, instead of a single-headed arrow, you’ll often see chemical equations use two single-sided arrows to reflect the state of equilibrium.
Now we’re indicating that not only are the hydrogen and oxygen combining to form water, but water is also decomposing to form hydrogen and oxygen.
Thermodynamics
The equilibrium for a reaction is not an arbitrary ratio of reactants and products. It is directly related to the temperature of the reaction and the Gibbs free energy, a thermodynamic property of the reaction.
While a thorough discussion of the Gibbs free energy is beyond the scope of this video, we’ll provide a brief introduction to enthalpy and entropy, the quantities that determine the Gibbs free energy.
Enthalpy
The change in enthalpy is the energy difference between the reactants and products. If the products are higher in energy than the reactants, the change in enthalpy is positive and the reaction is endothermic. If the products are lower in energy than the reactants, the change in enthalpy is negative, and the reaction is exothermic.
That energy is released, which is why some reactions, like combustion, are explosive. The change in enthalpy is often represented using a reaction coordinate, where the y-axis is energy and the \(x\)-axis shows the progression of the reaction.
The reaction of hydrogen and oxygen to form water is exceptionally exothermic and releases energy in the form of heat and light (think of the explosion of the Hindenburg!). We can conclude from this that the hydrogen-oxygen bonds of water are more stable than the hydrogen-hydrogen and oxygen-oxygen bonds. Conversely, when water decomposes to form hydrogen and oxygen, the reaction is endothermic.
Entropy
The entropy of a system reflects the number of possible configurations and increases when more configurations are made available. As the second law of thermodynamics tells us, the universe tends toward disorder, which means that only processes that increase the universal entropy are spontaneous.
How does this relate to our example? When the two hydrogen molecules and one oxygen molecule combine to form two water molecules, the total number of molecules is reduced from three to two. This, in turn, reduces the number of possible configurations (there are more ways to arrange 3 molecules than 2 molecules), which means the reaction entropy is negative.
Gibbs Free Energy
The Gibbs free energy takes both the reaction enthalpy and entropy into account and is the change in enthalpy minus the product of temperature and the change in entropy.
The Gibbs free energy can then be used to determine the preferred thermodynamic equilibrium of the system. The reaction will move in whatever direction necessary to achieve that equilibrium. So if there is too much reactant, more product will be generated and vice versa. This is how to determine the direction of the reaction.
Keep in mind, though the equilibrium is a result of the system’s thermodynamics, chemists can make adjustments to drive a reaction in a desired direction.
Reaction Kinetics
Now that we’ve covered the extent and direction of a reaction, let’s close with a discussion on the kinetics, or speed of the reaction, which is a completely separate subject.
A number of factors impact the rate of the reaction.
Collision Frequency
First of all, for a reaction to take place, the reactants need to be physically close enough to react. Returning to our example, the more frequently hydrogen and oxygen meet in space, the more likely a reaction is to occur- in other words, the rate of the reaction will increase.
Chemists can increase the number of collisions by either making the reaction vessel smaller or by adding more of each gas to the vessel. This way, we ensure that the reactants have the opportunity to react. Similarly, when the reactants are solid, increasing the available surface area of the solid increases the opportunities for reactions to take place.
Activation Energy and Catalysts
Next, remember we introduced a very simple reaction coordinate when discussing the change in enthalpy. However, reactions rarely proceed this simply. Intermediate steps are required to convert the reactants to products.
Consider our water formation. To form the hydrogen-oxygen bonds of water, we also have to break the H2 and O2 bonds, which takes energy! So a more accurate representation of the reaction coordinate shows a transition state with higher energy. The barrier this creates for the reaction is called the activation energy. The reactants cannot proceed to the products without sufficient energy to get over the activation energy.
Thus, for a reaction to occur, the reactants not only need to meet in space, they must meet in space with enough energy to overcome the activation barrier. If our reactants rarely have enough energy to achieve this, the reaction will proceed slowly. For example, though hydrogen and oxygen are both in the atmosphere, they are unlikely to react at those conditions because they lack the necessary energy.
So, chemists have two options. They can (1) provide the reactants with more energy such that they can overcome the barrier or (2) they can lower the activation energy. The first is achieved by providing energy to the reaction, typically in the form of light or heat, and the second can be achieved with the addition of a catalyst. Catalysts interact with the reactants to lower the energy of the transition state, thus lowering the activation energy and increasing the chance of reaction.
Review
With that, let’s wrap up with a review of what we’ve covered today.
We began with an introduction to the standard notation used to describe a reaction, the chemical equation. We then segued into a discussion on the extent and direction of the reaction, noting that most reactions reach equilibrium with constant concentrations of both reactants and products. The equilibrium is determined by the thermodynamic quantities of the reaction; the enthalpy, entropy, and Gibbs free energy.
And lastly, we considered what factors, and how we can alter them, impact the speed of the reaction.
Thanks for watching, and happy studying!