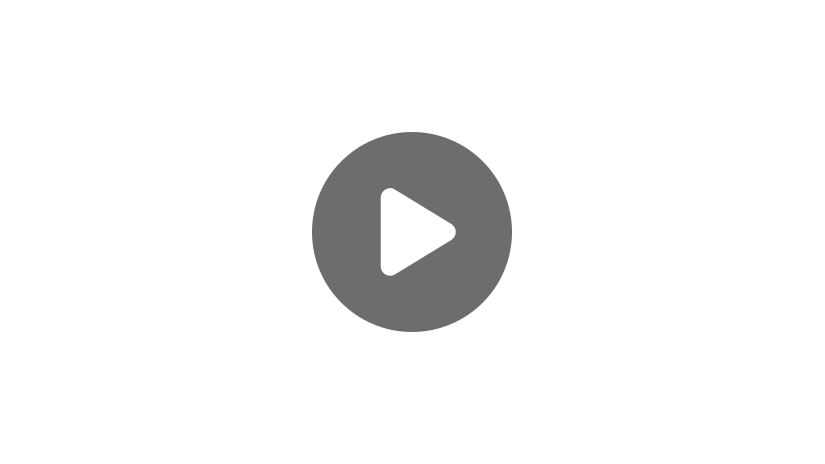
Hi, and welcome to this video on radioactivity! Today, we’ll be taking a look at what all is involved in the process, as well as the different types of decay that result from it.
Let’s get started!
What is Radioactivity?
So, let’s start with the basic definition. Radioactivity is the spontaneous process through which an unstable nucleus disintegrates, by way of the emission of particles or photons.
Radioactivity is categorized as a nuclear reaction not a chemical reaction, since the result is a completely different nuclear arrangement and not just a different compound.
Let’s break that definition down even further. Remember, the core of every atom is a number of neutrons (N) and a number of protons (Z) collectively called the nucleus. Radioactive decay is when an unstable nucleus breaks apart and emits the excess energy through photons or particles.
Nuclear Transmutation
Depending on the type of emission, the atom may undergo nuclear transmutation; in other words, it changes its elemental identity. This is actually what alchemists were hoping to achieve when they were trying to turn other elements into gold.
Nuclide vs. Isotope
A quick note on nomenclature. When discussing matters of nuclear physics, the term nuclide is often preferred over isotope. While both terms refer to specific compositions of particles in the nucleus, the term isotope refers to variations of a specific element, because it is focused on the number of neutrons only.
Whereas nuclide is used when discussing the results of nuclear reactions, because it draws attention to the number of protons and neutrons. As you can see here, the unstable nucleus is called the parent nuclide and the product is called the daughter nuclide. The daughter nuclide may be stable, in which case the reaction stops, or it may be unstable and proceed through further radioactive decay.
Nuclide Stability
Now, we tend to only think of heavier elements as being radioactive, like uranium and radium. And while it is true that many heavy elements are radioactive (we will discuss why this is the case later on), every element, even hydrogen, has the potential to be radioactive; it simply depends on the stability of the specific nuclide.
One measure of the stability of a nuclide is the half-life. The half-life of a nuclide is defined as the average time it takes for half of a given number of atoms of that nuclide to decay. The shorter the half-life, the more unstable the nuclide.
Nuclear Composition
Remember that within every element there are different isotopes (atoms with the same number of protons but a different number of neutrons). For example, hydrogen, in its most abundant form, has a nucleus of one proton. However, deuterium has one proton and one neutron, while tritium has one proton and two neutrons.
All three of these nuclides are isotopes of hydrogen, meaning they have the same Z value but different N values. In chemical reactions, these three isotopes generally behave identically; however, only tritium is radioactive.
This tells us that radioactivity is related to the specific composition of the nucleus and, more specifically, to the number and ratio of protons and neutrons. This also highlights that any element can be radioactive given adjustments to the nucleus.
Factors Affecting Nuclear Stability
Exactly why certain isotopes are unstable is complex and a full explanation is well beyond the scope of this video, but there are some trends we can discuss.
First, heavier elements are more likely to be radioactive than lighter elements. In fact, all nuclides after bismuth, the 83rd element, are radioactive. This means that there are no stable isotopes for these elements. We can make some sense of this if we take a moment to think about the nucleus and why it might be unstable in the first place.
To form a nucleus of any element other than hydrogen, we have to cram protons into a teeny, tiny space. This results in a large repulsive electrostatic force, which doesn’t sound stable at all. So what keeps the nucleus together in the first place if the protons are repelling each other? The repulsive electrostatic force is counteracted by the strong nuclear force, which exists between protons and neutrons, and stabilizes the nucleus.
While the strong nuclear force is indeed very strong, it is only strong over very small distances—this is why nuclei don’t collapse into each other. So as a nucleus gets larger, the repulsive electrostatic force begins to match the strong nuclear force.
In other words, the force pushing the particles away from each other becomes similar in magnitude to the force keeping the particles together. As a result, large nuclei are unstable and spontaneously break apart. This also puts a theoretical cap on the size of the periodic table as heavier elements become less stable.
The second reason why nuclides may be unstable is a high or low N/Z ratio. Generally, nuclides with N/Z ratios close to 1, or slightly higher than 1 as Z increases, are stable. When a nuclide is very neutron- or proton-rich, they tend to be unstable.
Given what we’ve just discussed about the repulsive electrostatic force between protons, it should come as no surprise that proton-rich nuclides are unstable. A more complex explanation is needed for the mechanisms associated with neutron rich nuclides, so for now, just know that an excess of neutrons is also unstable.
It is important to note that nuclei with even N and Z values tend to be stable. Of all the stable nuclides, 260 out of 264 have either even numbers of protons or neutrons or both. There are also certain values of N and Z, the so-called magic numbers, which are exceptionally stable. This leads physicists to theorize that, similar to electron energy levels, there are nucleon energy levels.
Modes of Radioactive Decay
Now that we have a sense of why nuclei can be unstable, let’s consider how they decay. When a nucleus breaks apart, there are three modes of decay- alpha, beta, and gamma, which are named for the type of emission associated with each.
Alpha Decay
Alpha decay is the emission of an alpha particle, which is two protons and two neutrons.
This particle is a helium nucleus. This, of course, results in the nuclear transmutation of the nuclide, with Z decreasing by 2. Alpha decay is characteristic of the heavier elements past bismuth, which sort of makes sense, since those nuclides are much too heavy to be stable, and thus, they make a large change to the structure of the nucleus. For example, radium undergoes alpha decay to yield radon.
Note that rather than writing He2+, nuclear physicists prefer to use \(\alpha\), \(_{2}^{4}\alpha\), or \(_{2}^{4}He\), since the focus is on the number and composition of the nucleons and not on the charge of the particle.
Beta Decay
Beta decay includes three different types of decay, \(\beta^{-}\)(electron) decay, \(\beta^{+}\)(positron) emission, and electron capture, but to keep things simple, we’ll just be looking at \(\beta^{-}\) decay today. \(\beta^{-}\) decay is the release of energy through the emission of a high-speed electron, or as it is known in nuclear physics, a \(\beta\) particle. This electron shoots out at a very high speed due to the conversion of a neutron into a proton.
This is an electron from the nucleus, not an orbital electron, which is why it’s important to use the \(\beta^{-}\) notation to keep them straight. This equation is showing our neutron turning into a proton, which results in the emission of the \(\beta^{-}\) particle. We can gather from all of this that -decay results in nuclear transmutation, as it is accompanied by a +1 change in Z. For example, carbon-14, which is used in radiocarbon dating, decays to nitrogen-14 through \(\beta^{-}\)decay.
Gamma Decay
Lastly, let’s talk about gamma decay. Gamma decay involves the release of high-energy gamma radiation. This often happens just after alpha or beta decay when the resulting daughter nuclide is still high-energy and relaxes by releasing gamma radiation.
Gamma rays are photons, so they carry no mass and thus do not affect the composition of the nuclide. All three types of radioactive decay can be dangerous to organic life forms, potentially breaking up DNA and damaging cells enough to kill them or mutate them in ways that could lead to cancer. However, in spite of the danger, some radionuclides can be used in helpful ways within the body. We can use them as tracers for material flow, biological reaction pathways, or even medical diagnostics.
Though there are naturally occurring radionuclides, we also produce them using particle accelerators, in which stable isotopes are bombarded with particles to produce new radionuclides. Using this method, we have also discovered new chemical species that would not exist naturally.
Review
Okay, before we finish, let’s go through a quick recap!
Radioactivity is the spontaneous breakdown of an unstable nucleus. And, while heavy elements past bismuth are always radioactive, all elements can be radioactive depending on the specific composition of the isotope. This results from an imbalance of the forces pushing apart and pulling together the nucleus. And finally, the type of decay a nuclide undergoes (alpha, beta, or gamma) is related to why it is unstable, e.g. too large, too many protons, too many neutrons, etc.
I hope this review was helpful! Thanks for watching, and happy studying!