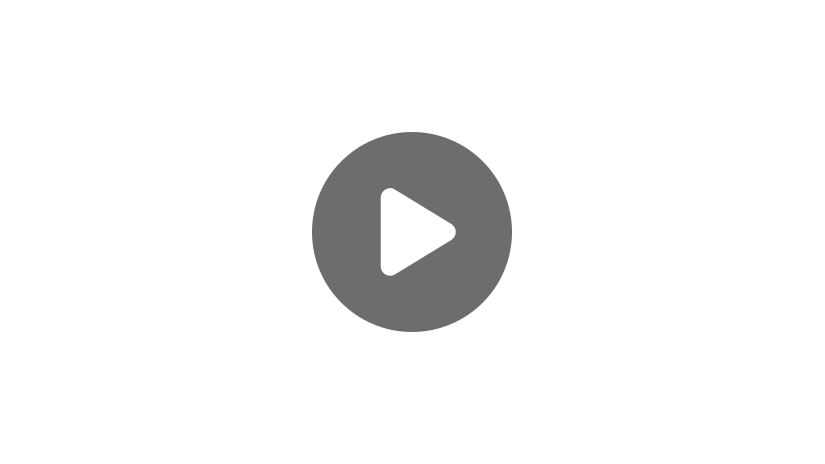
Hi, and welcome to this video on chromatography!
Today, we’ll cover the general process of chromatography, discuss the different chemical and physical properties used in separations, and look specifically at the instrumentation used for gas and liquid chromatography.
Hopefully by the end of the video, you’ll not only understand the theory of chromatography, but also when to use it and why it is a critical tool for chemists.
What is Chromatography?
Chromatography serves to separate, identify, and quantify multi-component mixtures. As such, it is undoubtedly the most powerful tool for an analytical chemist.
There are many types of chromatography, including paper, thin-layer, gas, and liquid. In fact, even if you’re not a chemist, you’ve probably accidentally done some simple chromatography. If you’ve ever clumsily spilled water on some marker ink, you may have noticed the ink separating into its parent colors. That was chromatography! You took a complex mixture of ink and split it into its component pieces.
We’re going to focus on gas and liquid chromatography today, but the same principles are in action for paper and thin-layer as well.
The Chromatography Process
To begin, let’s look at a simplified chromatography process with a visual aid.
Consider a mixture of blue and red particles. We’re going to separate them using chromatography. To start with, we have a column, perhaps made of metal or glass, filled with a stationary phase. By that, we literally mean stationary, as in the chemicals inside do not move. They stay in place inside the column, even when we introduce our mobile phase.
The mobile phase (a fluid of some kind, liquid or gas) passes through the column but doesn’t bring the stationary phase with it. Now, we add our mixture of blue and red particles, which is called the solute in chromatography chemistry, to the top of the column.
The flowing mobile phase brings the particles into the column—where things get interesting. The blue and red particles now must decide which they like more, the stationary phase molecules or the mobile phase molecules. And yes- we’re describing the particles as if they are some sort of sentient beings, but we’ll follow up with the actual chemical and physical reasons behind their choices later.
The red particles are more attracted to the stationary phase, so are more frequently absorbed by the stationary phase. In other words, red particles are more likely to get hung up in the stationary phase than blue particles. This means that more blue particles are in the mobile phase, which is continually flowing, so the blue particles travel down the column at a faster rate than the red particles.
Eventually, a band of blue particles will leave the column with the mobile phase and a band of red particles will leave the column at a later time. Boom! Separated!
We can represent the results of our column chromatography using a chromatogram.
Time is on the \(x\)-axis and the detected response, which can be correlated to the amount of solute, is on the \(y\)-axis. We track the amount of solute coming out as a function of time, time zero being when the solute was added to the top of the column.
So, for our example, we have a chromatogram showing a blue peak at time \(t_{4}\) and a red peak at time \(t_{5}\). If the right instrument is used and calibrated properly, then the area under the peak is directly related to the quantity of each solute that was in the original sample.
In this simplified example, we detected the particles with our eyes- we could see the blue and red particles in the mobile phase when they left the column. This won’t always be the case, and often isn’t, so we usually need more sophisticated methods of detection.
But before we move on to the specifics about the different chromatography instruments, let’s delve more deeply into how the particles are separated- because this is the key to successful chromatography. We must create an environment in which the components of our mixture move at different speeds. So what physical and chemical factors impact the speed at which particles move through a column?
The underlying chemical or physical mechanism that separates the particles lend their name to the different types of chromatography: adsorption, partition, ion-exchange, molecular exclusion, and affinity. We’ll give a quick overview of each.
Types
Adsorption
Adsorption chromatography, sometimes referred to as liquid-solid chromatography, uses a solid stationary phase on which solute is adsorbed through intermolecular interactions like van der Waals forces and hydrogen bonding.
Solute not adsorbed by the stationary phase is carried along with the mobile phase and has a shorter retention time.
Partition
Partition chromatography uses a solid stationary phase coated with a thin film of liquid, which is immiscible with the mobile phase. During this type of chromatography, solute equilibrates between the liquid stationary phase and the liquid mobile phase.
Ion-Exchange
Ion-exchange chromatography uses ions covalently attached to the stationary phase to attract solute through electrostatic forces. This method is typically used when separating charged molecules.
Molecular Exclusion
Molecular exclusion chromatography separates solute particles based on their size, which makes this a physical, and not chemical, form of separation.
The stationary phase is filled with tiny pores, which larger particles can’t move through. Thus, larger particles have a much shorter path through the column than smaller particles, and leave the column first.
Affinity
Lastly, affinity chromatography is the most selective form and is specifically developed for a single molecule. This is achieved by covalently bonding a molecule to the stationary phase that has a strong interaction with only one solute molecule.
This is often used for biological mixtures, where an enzyme or antibody is affixed to the stationary phase. The protein to which those biomolecules are attracted can be separated from a mixture of thousands of proteins.
So those are the five primary ways in which the solute interacts with the stationary phase. Even if you don’t remember the specifics of each, what’s important to remember is that because the particles in the mixture have different interactions with the stationary phase, they move through the phase at different rates.
Using Gas vs. Liquid
Another critical choice in selecting the best chromatographic method is whether to use gas or liquid for your mobile phase. This is actually the categorization most often used for chromatography because this will determine the instrument required, a gas chromatograph (GC) versus a liquid chromatograph (LC).
These are exceptionally powerful instruments and are often automated to run hundreds of samples a day. The general principles of each are essentially the same as our simplified column example earlier. The method selected, GC or LC, depends largely on the volatility of the solute (how readily the solutes vaporize), as gas chromatography requires the solute to be in the gas phase.
We’ll look at a general schematic of each instrument and point out some key features as we go. Let’s start with gas chromatography.
Gas Chromatography
The first step of gas chromatography is the vaporization of the sample.
To start, the mixture is injected into the instrument and immediately heated. Solute particles are vaporized and swept into the column with a carrier gas. The entire GC column is in an oven and the temperature is programmed to increase over the course of the separation.
As the column gets hotter, the solutes spend more time in the gas phase, rather than attached to the stationary phase, and thus, pass through the column at an increased rate. Solutes exit the column and reach a detector, which transmits a signal to a computer that displays the signal as a function of time.
The exit order of solutes typically follows the order of volatility, meaning that the most volatile solute has the shortest retention time and the least volatile solute has the longest retention time.
Liquid Chromatography
Now let’s switch over to liquid chromatography.
These samples aren’t volatilized. Instead, they’re added in liquid form to the top of the column and the liquid mobile phase carries them through the column. LC columns are usually much shorter and wider than GC columns and don’t need to be heated, although most modern LC instruments have the option. The solute separates in the column and the mobile phase carries them to a detector, which transmits the signal to a computer that displays the signal as a function of time.
Detectors for LC are often UV/VIS or mass spectrometers. LC procedures often call for the composition of the eluent (the mobile phase liquid) to be adjusted. The properties of the eluent significantly alter how long solutes stay in the column and can be adjusted to slow down or speed up the process.
It is essential to find a balance in solute speed. If the solutes travel too quickly, then they won’t separate enough, but if they travel too slowly, then the signal may be too weak to be detected.
Chromatography Uses
GCs and LCs are often used for analytical purposes, meaning scientists are analyzing the sample—separating, identifying, and quantifying the different components. Analytical chromatography is an extremely powerful tool and has many important applications. For example, checking the quantity and purity of drugs or measuring the amount of a protein in your blood.
It’s important to note that chromatography cannot be used to provide the exact chemical identity of a compound. Based on the retention time of the substance, we can infer some things about it but can only confirm its existence and not establish the molecular structure.
A lot of the time, chemists already know the identity of the solute molecules and are using chromatography to isolate each component for analysis. But if the solute is unknown, chemists must use other instrumentation, like mass spectrometry or NMR, after separation to determine the structure.
Liquid chromatography can also be used for the preparation of materials, often on much larger scales than when done for analytical purposes. In this kind of preparative chromatography, scientists know their targets and use chromatography to purify them for future use.
Hopefully, through all this, you’ve picked up that, while the underlying principles of chromatography are fairly straightforward, there are A LOT of ways to adjust the methodology: different stationary phases, mobile phases, temperature gradients, eluent gradients, different detectors and on and on.
Quantifying Success
Lots of research and effort is put into creating chromatography methods that successfully separate the solutes in a complex mixture. Which brings us to our final point—How do we quantify the success of a chromatographic method? For that, we’ll need to return to our chromatogram.
The most important result of chromatography is that the solutes are sufficiently separated. Let’s look at a few different chromatograms to understand this further.
This is an ideal chromatogram. The retention times for the two solutes are very different and the peaks are narrow such that they are well separated. Now consider these two suboptimal chromatograms:
Here, the retention times are too similar and the peaks overlap.
Here, the peaks are too wide for the difference in retention times. Methods must be finely tuned to achieve satisfactory separation between solute peaks but also be economical and timely.
With that, we’ll wrap up today’s video with a couple of quick review questions.
Review Questions
1. The underlying chemical or physical mechanism that separates the particles lend their name to the different types of chromatography. What are the 5 mechanisms that we discussed in this video? Pause the video if you need some time to think.
2. Which of the following is NOT true of gas chromatography?
- GC columns are usually much shorter and wider than LC columns.
- The first step is the vaporization of the sample.
- The temperature of the column is programmed to increase over the course of the separation.
That’s all for this review of chromatography! Thanks for watching, and happy studying!