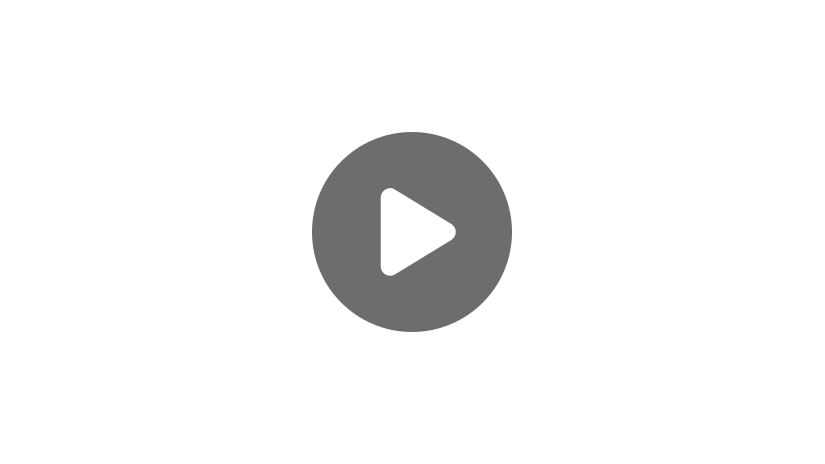
Hi, and welcome to this video on single-replacement and combination reactions, two of the five main categories of chemical reactions. We’ll define each, cover how to recognize them, predict their products, and go over some examples.
Single-Replacement Reactions
Let’s begin with single-replacement reactions.
The general form of a single-replacement reaction is this [image at 0:28] where an aqueous solution of A cations and B anions reacts with C in its elemental form. Here, we’ve shown C replacing the cation, A, to form aqueous CB, and producing A in its elemental form, which makes this more specifically a cation replacement reaction. Whether this reaction proceeds depends on the relative reactivity of A and C.
We’ll cover relative reactivity in a minute, but first I want to point out that we could also have written the reaction showing C replacing the anion, B, producing aqueous AC and elemental B. This is an anion replacement reaction.
Again, whether this happens will depend on the relative reactivity of B and C.
Oxidation-Reduction
Notice that in either scenario electrons are moving between species. For example, in the cation replacement reaction, A gains electrons and C loses electrons. This means single-replacement reactions are by definition oxidation-reduction reactions.
To drive this point home, we’ll assign oxidation states in some of our examples to follow the flow of electrons in the reaction.
Cation Replacement Example
Let’s dive in now and look at an example of a cation replacement reaction where solid iron reacts with a solution of copper nitrate to form solid copper and iron nitrate.
Comparing the chemical equation to our generic equation for single replacement, A is a copper ion, B is a nitrate ion, and C is solid iron. As the reaction proceeds, iron replaces copper cations in solution and, in turn, copper ions come out of solution and elemental copper is formed.
Remember that metals typically form cations, so we can expect to see metals as the active species in cation replacement reactions. Note that the nitrate ions don’t really participate in the reaction, except to balance the positive charge of the cations. Consequently, the nitrate ions are spectator ions in the replacement reaction.
Assigning Oxidation States
Now let’s assign the oxidation states for this reaction.
Iron begins with an oxidation state of 0 and ends with an oxidation state of +2, so it has lost electrons. This means iron was oxidized. Copper picks up those electrons, going from an oxidation state of +2 to 0, which means it was reduced.
Reactivity
We can use this information to help understand why the reaction proceeds in the first place.
Iron loses electrons, which tells us that iron is the stronger reducing agent of the two metals (remember, that because iron was oxidized, it acts as the agent that reduces). In other words, the system is more stable when iron gives up its electrons and carries the positive charge while copper is in its elemental state. But what factors contribute to the strength of a reducing agent?
Without going into too much detail, the strength of a reducing agent is related to the low ionization energy and small electron affinity of the element. In other words, how easy is it to remove a valence electron and how much does it “desire” an additional electron. Generally, metals, especially those on the left side of the periodic table, have low ionization energies and small electron affinities and thus, readily form cations.
Based on the results of our example reaction, we can intuit because iron is oxidized that it is easier to remove electrons from iron than it is from copper. And indeed this is true. Ultimately, ionization energies and electron affinities can be understood by the electron configurations and the effective nuclear charge of the elements.
Activity Series of Metals
If this all feels overwhelming, don’t worry. We’re just providing a bit of additional information that hopefully makes this feel more intuitive. The good thing is, you don’t actually have to remember the relative ionization energy and electron affinities of every metal, because chemists have helpfully compiled an activity series of metals, showing the relative reactivity of metals.
This list uses the metal’s ability to displace hydrogen in an aqueous solution as a reference point, but again, can be understood on an atomic level by considering the ionization energies and electron affinities of each element. Any metal listed above hydrogen in the activity series can displace hydrogen (under varying conditions) and any metal listed below hydrogen cannot displace hydrogen.
So for example, when you add solid lithium, the most reactive metal, to water, it readily gives up its valence electron and replaces the hydrogen in water, producing lithium hydroxide and hydrogen gas (notice that this is a single-replacement reaction!)
Conversely, when you add gold to water or even an acidic solution, no reaction proceeds (which is good, otherwise, gold jewelry wouldn’t last too long!). This is because gold is listed below hydrogen in the activity series.
Predicting Reaction Direction
Along with telling us about the metal’s ability to displace H2, the activity series also allows us to predict whether a replacement reaction will occur between two metals. Only a more reactive metal, one that is higher up the list, can replace the reactant metal.
Notice that this is consistent with our example reaction. Iron is indeed higher than copper on the activity series. Furthermore, notice that we can also intuit from this that the reverse reaction would not happen.
Keep in mind that the boundaries and exact order depicted in the activity series are not hard and fast. The order may change depending on the type of reaction, but for the examples we’ll cover today, you can reference this activity series to predict whether a reaction will take place.
Combination Reactions
Elemental starting materials can also combine to form covalent compounds. Take for example the Haber-Bosch process, which combines nitrogen gas and hydrogen to form ammonia.
Combination reactions can also include compounds in addition to elemental reactants. For example, sulfuric acid is formed in the atmosphere from sulfur trioxide and water.
Notice that the reverse of a combination reaction is a decomposition reaction, where a single species splits into two or more components.
While you may be tempted to guess that all combination reactions are exothermic because a new bond is formed, which is true most of the time. There are some endothermic combination reactions. For example, the decomposition of hydrogen peroxide is exothermic.
Also, notice that all combination reactions are not necessarily redox reactions. Take a second to look back at our examples and check. You’ll find that when the reaction included a pure element, redox occurred, but when the reactants were compounds, as in the formation of sulfuric acid, it was a non-redox combination reaction.
Furthermore, unlike the activity series for the single-replacement reactions, we do not have a template to guide us in deciding whether a combination reaction will proceed. To predict the direction and product of a combination reaction, we will typically require a more intimate knowledge of the reactants as well as the thermodynamic properties of the reaction.
So keep in mind that combination reactions encompass a wide range of chemistry and it’s not easy to make generalizations about them like it was for single-replacement reactions.
Practice
Finally, let’s wrap up with some practice. Look over the following reactions and assign them as a single-replacement reaction or a combination reaction. If the reaction is a single-replacement reaction, predict whether it would proceed in the direction shown. And if you’re feeling up to a challenge, identify which are redox reactions.
Ready? All right, here are the reactions: [reactions onscreen at 8:50] If you’d like to try your hand at them, go ahead and pause the video and work through the problems.
Finished? Here are the correct assignments for each reaction: [onscreen at 9:20]
Thanks for watching, and happy studying!